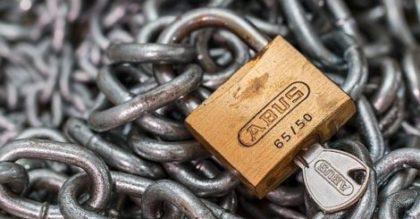
As you read this, millions of people around the world are making antibodies against SARS-CoV-2. None of those people were born with a gene for those specific antibodies. Yet in just a couple of weeks following exposure to the virus–or now, one of its proteins via a vaccine–their immune systems can make antibodies that bind tightly and very specifically to the outside of the coronavirus, inhibiting its ability to infect their cells. The close and unique match between antibody and antigen has been compared to the fit of a key to a lock. While inert macroscopic metal objects don’t fully capture the Brownian mosh pit of molecular dynamics, keys and locks can be a helpful starting point for understanding why each virus requires a tailored immune response. And that unique fit is achieved via an evolutionary mechanism of selection and inheritance with variation.
I was a senior in high school when the World Wide Web started to take off. I could only surf in the school library; our PC at home was still running DOS. I would type in URLs from an Internet yellow pages and bask in the glory of scrolling banners, star field backgrounds, and three digit page visit counters. One of my early fascinations was the MIT Guide to Lockpicking. I never came remotely close to actually picking a lock, never even figured out how you’d get the required tools. But the allure of supposedly clandestine knowledge of what cartoon characters were meant to be doing with those bobby pins was all I needed to print out a copy and pore over it repeatedly. Well, that and the satisfaction of finally understanding how locks worked after using them for a decade.
If you’re not familiar, the lock contains a series of spring-loaded pins. At rest, those pins keep the handle or deadbolt from turning. The easiest way to get the lock to turn is to have the right key, one with notches that lift those pins to the necessary heights to align grooves that will allow the handle by. An alternative involves trying to feel out the right height, one pin at a time. The lock can provide feedback on the appropriate heights so that you don’t have to stumble on all of them at the same time. Obviously I don’t endorse trying the process (and I’ve almost certainly forgotten relevant particulars), but I wanted to illustrate different kinds of approaches to solving a problem. We can think of this approach as an iterative process of exploring and feedback.
Exploration with feedback covers a whole range of problem-solving methods. You try a solution, find out how well it works, make adjustments, and try again. To a first approximation, evolution works this way. In the case of a viral infection, the problem is making a protein (an antibody) that complements the particular shape and chemical properties of a viral protein so that tight and specific binding is achieved. Your body starts with the antibodies it was born with genes to make. Different B cells (a particular kind of white blood cell) each use a different antibody gene to make a different antibody. Most likely, none of those antibodies match closely enough to prevent infection. But those B cells get signals to grow and divide in proportion to how closely their antibody matches. They each make more copies of themself and their antibody gene. During that copying step, mutations are introduced, even more than happen in typical DNA copying to speed things along. If one of the descendant B cells has a mutant antibody gene that binds a little better, that cell will get even stronger signals to divide further. This process iterates until an antibody that binds tightly and specifically is produced.
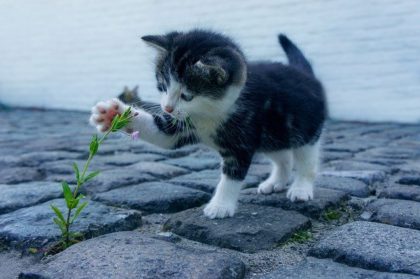
In this case, mutations are the means of exploring. The B cells are exploring the (abstract) space of all possible antibody genes to find one that works. That space is incomprehensibly vast. So the exploration is coupled with feedback to find a solution more quickly. In this case, feedback comes from testing the binding and sending growth signals proportional to the strength of the binding. Even if some of the B cells with antibodies that are poorer fits also get some growth signals, over time the cells with the best fitting antibodies will get the most signal and divide the most, so their antibodies will be the most prevalent among the descendants. It can take time for that advantage to play out, as well as the time needed for multiple rounds of mutation, which is why a couple of weeks are needed (hence the gap between doses in two-dose COVID-19 vaccines). Nevertheless, the process plays out fairly reliably, as evidenced by the 95% efficacy of the COVID-19 vaccines. When all is said and done, most everyone who gets the vaccine will wind up with B cells that have an antibody gene they were not born with, that forms a strong protein-protein interaction with a virus no one had ever heard of when they were born.
Now, the viability of an evolutionary process to produce neutralizing antibodies does not prove that all organisms share common ancestry or that descent with modification explains all biological diversity. It simply illustrates that evolution is a competent problem-solving method at least for some problems, and one that can be implemented biologically. More than the natural history of what species begat what other species, more than the still-fascinating details of gene duplication and hybridization and niche construction and all sorts of evolutionary biology, the simplicity and effectiveness of the problem-solving method at the center of evolution is what I find compelling about the topic.
That’s not to say evolution can solve any and every problem. Abstractly, we can prove that no algorithm works well on all possible problems. Experimentally, we can see that some biological features takes longer to evolve than others, if they can evolve at all. Clinically, we know that exploration can take steps in unfruitful directions; for example, introducing mutations to try to find good antibodies can sometimes instead cause B cells to become cancerous. As we try to correct misunderstandings about evolution seeming less than it actually is, we don’t want to overcorrect and think more highly of it either.
Finally, as we learn more about evolutionary biology in future posts, we’ll see the scope of the biology involved is broader and richer than can be neatly summarized by exploration and feedback, or DNA copying mutations and amplified reproduction. Not every feature of every organism is there because it was selected for, and selection is not the only factor in determining what features persist. And there are other forms of exploration besides individual copying mutations. Still, before we get into all the amazing details, I wanted to start with a zoomed out view of evolution as a problem-solving process. I think that’s a helpful launching point, and I think it helps explain why we will be spending time on computer simulations as well as biology, starting next week.
About the author:
Andy has worn many hats in his life. He knows this is a dreadfully clichéd notion, but since it is also literally true he uses it anyway. Among his current metaphorical hats: husband of one wife, father of two teenagers, reader of science fiction and science fact, enthusiast of contemporary symphonic music, and chief science officer. Previous metaphorical hats include: comp bio postdoc, molecular biology grad student, InterVarsity chapter president (that one came with a literal hat), music store clerk, house painter, and mosquito trapper. Among his more unique literal hats: British bobby, captain's hats (of varying levels of authenticity) of several specific vessels, a deerstalker from 221B Baker St, and a railroad engineer's cap. His monthly Science in Review is drawn from his weekly Science Corner posts -- Wednesdays, 8am (Eastern) on the Emerging Scholars Network Blog. His book Faith across the Multiverse is available from Hendrickson.