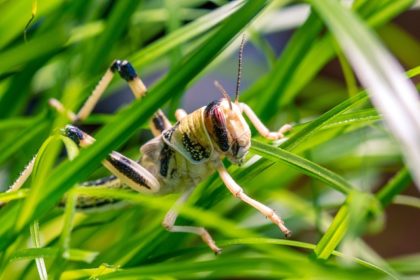
In the last installment of Three Chromosomes in a Trench Coat, we learned about gene expression and epigenetics. This episode takes a look at how changes in gene expression can drastically change the phenotype of an organism and help it survive in a changing environment (Spoiler alert — at the root they are the same processes). In many cases changing the expression of a few genes allows organisms to adapt to a change in the environment. For example, when the environment gets hotter, an insect (clam, spider, anemone, worm, etc.) a can upregulate (a.k.a. increase the expression of) heat shock proteins. Heat shock proteins (or Hsps) protect other proteins from being damaged by the warm temperatures. Similarly, when temperatures cool, upregulation of proteins that produce glycerol, and other sugar-alcohols, prevent internal tissues from freezing. Even these moderate changes in gene expression improve an organism’s fitness (a.k.a. the number of offspring contributed to the next generation). But there are also a number of organisms that, in the face of a changing environment, can alter the expression of hundreds of genes to produce an entirely different phenotype. Here are 3 examples that I think are really cool.
Daphnia are one example of extreme phenotypic plasticity. These microscopic crustaceans (relatives of shrimp and lobsters) undergo extreme morphological changes (a.k.a. changes its appearance) when predators are present. In response to chemicals produced by the fish that eat them, Daphnia can develop neck-teeth, a spikey helmet and tail spikes that make them more difficult to eat. The timeline for this change isn’t clear, but at least in some species it takes approximately 48 hours from the time that the chemical to is detected until the transformation begins to appear. Some of the genes that are involved in changing Daphnia are involved in producing hormones and chemicals that serve a signals throughout the body. Some other genes are involved in metabolism. Many other genes are involved in determining the morphology (a.k.a. physical appearance) during development and are similar to genes used in the development of limbs and other physical structures in other animals, including humans (Miyakawa, et al., 2010).

When morning arrived, the east wind had brought the locusts. 14And the locusts swarmed over the whole land of Egypt, settling in dense swarms from one end of the country to the other. It was the worst locust plague in Egyptian history, and there has never been another one like it. (Exodus 10:13-14, NLT)
Another fascinating example of phenotypic plasticity is the locust. Locusts are a type of grasshopper that can take either a solitary form or a gregarious, swarming form. Individuals raised in isolation or in low densities with out too many near neighbors are solitary. When conditions get too crowded, individuals become gregarious and swarm like in the Egyptian locus plague in the Bible or the swarm in Africa in 2020. The phase change from solitary to swarming occurs when individuals become crowded in response to a physical touch on their hind legs. A change in behavior occurs within a few hours after a touch threshold has been reached. Changes in appearance and physiology take longer (Simpson et al., 2001).
The change from solitary to gregarious forms happens in response to serotonin and other hormone signals that initiate a downstream change in the expression of more than 500 genes. Locust phase change is also a maternal effect, which means that the mother’s history and environment is passed on to her offspring via epigenetic mechanisms. A solitary-phase female that is crowded when she reproduces has offspring that are gregarious. A gregarious female who is isolated when she reproduces has offspring that are the solitary form (Simpson and Sword, 2008).
The last example is near and dear to my heart — so much so that it’s the focus of my research program at The Ohio State University. I think that adaptations that allow insects to live in almost every habitat on Earth — including Antarctica! — are really cool. Many insects are able to survive seasons when the environment is inhospitable by entering a state of suspended animation (a.k.a. dormancy or diapause) that is characterized by slowed development, reduced metabolism, and increased tolerance of cold, dehydration, and oxygen limitation. In North America and other temperate climates insects enter diapause during the winter. Insects in the tropics can also enter diapause, but they are dormant during the dry season. This strategy provides insects with added protection against the challenges of winter, but it also allows populations of insects (all the insects of a species in the same geographic area) to synchronize the times when they are active and reproducing with seasons when there is a lot of high quality food available.
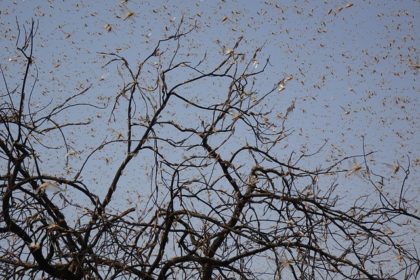
Most insects have multiple generations per year, and only one generation enters diapause. This means that diapause is a phenotypically plastic, alternative developmental pathway since it is one of two possible, developmental trajectories carried in the genome. The mechanics of switching from a developmental pathway without diapause to a trajectory with diapause is an interesting puzzle that scientists have been studying for several decades. During the last several years my colleagues have discovered that changes in gene expression are behind the switch in developmental trajectories. Some interesting genes that are involved in diapause include genes that code for parts of the circadian clock that measures daylength. In addition to measuring the number of hours of light (or dark) per day, the circadian clock it tied to metabolism. Using a technique called RNA-interference, it is possible to turn off genes known as “period” and “timeless”. In cabbage beetles and mosquitoes, turning off one of these genes alters the amount of fat that accumulates in the bodies of female cabbage beetles and mosquitoes (Meuti et al., 2015; Zhu et al., 2019). The amount of fat that accumulates and is stored in an insect that enters diapause is important because insects need to have enough energy to survive through the winter and restart development in the spring. Some other interesting genes that are involved in diapause include lipases, that break down fats so they can be used for energy, and the heat shock proteins we discussed earlier. Insulin and dopamine are genes that are important for regulating metabolism in diapause are also important for humans. My research shows that epigenetic mechanisms, especially small RNAs that regulate protein production, are likely involved in diapause. Ongoing work will, hopefully, reveal more about the relationship of epigenetic mechanisms.
I hope that so far this series has been instructional and provided some information about what a phenotype is, how it comes about, and how phenotypic plasticity can help organisms survive. Stay tuned for the final installment of this series where we will wrap up the discussion. Please feel free to leave comments about anything you have questions about or would like to see covered in more detail.
Dr. Julie A. Reynolds is a Research Scientist at The Ohio State University in the department of Evolution, Ecology, and Organismal Biology. She studies insect physiology and biochemistry with the goal of learning how animals adapt to extreme environments and survive changes in climate. In addition to writing for the Emerging Scholars Network, she is actively engages in discussions about science and faith as a Sinai and Synapses Fellow.