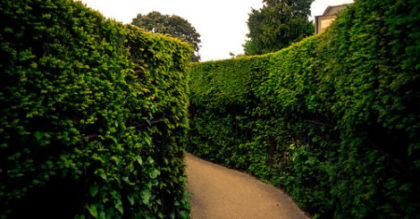

Perhaps you recall The Martian, the story of Mark Watney, an astronaut stranded on Mars trying to survive long enough to be rescued. His journey home involves driving for weeks in a solar-powered rover from his mission location to the launch site for the next mission’s return trip. There’s a part in the book (but not the movie) where a dust storm threatens to slow him down enough to miss his rendezvous. He needs to go around it, but he doesn’t know where it is or where it’s moving. In general, he wants to move towards where the dust is thinner (and thus blocks less electricity-generating sunlight), but the storm is so big that in any local area it’s pretty much equally thick in all directions. He needs a bigger-than-human scale perspective on his environment.
It turns out that individual cells face a similar challenge on a regular basis. Some cells need to move around, whether they are unicellular organisms in search of more food or cells of your immune system looking for an infection. The typical explanation for how they know where to go is chemotaxis. Maybe you’ve heard of phototaxis in plants, where they turn or grow towards a light source? Well, chemotaxis is like that but for chemicals. For example, an infected tissue might make a chemical called a cytokine to signal to the rest of the body that it is infected. The concentration of that cytokine will be highest at the source, the infected tissue, and will get lower the farther the cytokine diffuses away from that source. The cells of the immune system that need to infiltrate the tissue to respond to the infection just have to move towards higher concentrations of the cytokine. Simple enough, right?
Well, maybe not. If the concentration of the cytokine changes fast enough over a short distance, then the traveling cell may be able to sense a difference in concentration between one of its sides and another and move toward where it is higher. But if the concentration changes that much that quickly, it will also fairly quickly go to zero, meaning that it only works when the total distance that needs to be traveled is relatively small. This simple version also works best if the cell needs to travel in a straight line, but infected cells may be located in tissues or organs with structures that don’t allow for straight line travel. How does a cell know what to do if it comes to a fork in the road?
Watney’s solution to the problem was to drive substantial distances and take measurements at widely separated points to get a storm-scale view of the changes in dust concentration. This would be expensive, both in time and energy, for a cell responding to an infection. Plus it would also need a way of remembering the concentrations at different places and then computing a path from the various measurements, a challenge that may exceed the information processing capacity of an individual cell. Instead, what cells do is break down the chemical they are following so that there is less of it in the immediate area. Then as more diffuses from the source, the concentration will increase first in the direction the cell needs to move. This is called self-generated chemotaxis, because the moving cell is generating the local gradient to follow. And so a cell can decide where to go even if the chemical concentration initially looks the same all around it and without having to poke around first.
At least, that is the emerging model of how cells solve the problem. To test that model, scientists built little mazes for amoebae and mouse tumor cells to navigate. They also built mathematical models of self-generated chemotaxis to predict how the cells would perform in maze running if self-directed chemotaxis was their primary navigational procedure. Both types of cells were able to solve mazes, meaning move from start to end (where there was a chemical source) and make the correct decisions at branching points more often than expected from just guessing. And the dynamics of this behavior agreed with the math, suggesting self-generated chemotaxis is the mechanism involved. Below, you can watch some simulation results and some actual cells in the maze.
Elaine Eckland will be joining us for a conversation about common values in science & faith conversations, on Tuesday September 15th at 1pm EDT. Check out the details below and register here.
Andy has worn many hats in his life. He knows this is a dreadfully clichéd notion, but since it is also literally true he uses it anyway. Among his current metaphorical hats: husband of one wife, father of two teenagers, reader of science fiction and science fact, enthusiast of contemporary symphonic music, and chief science officer. Previous metaphorical hats include: comp bio postdoc, molecular biology grad student, InterVarsity chapter president (that one came with a literal hat), music store clerk, house painter, and mosquito trapper. Among his more unique literal hats: British bobby, captain’s hats (of varying levels of authenticity) of several specific vessels, a deerstalker from 221B Baker St, and a railroad engineer’s cap. His monthly Science in Review is drawn from his weekly Science Corner posts — Wednesdays, 8am (Eastern) on the Emerging Scholars Network Blog. His book Faith across the Multiverse is available from Hendrickson.
Leave a Reply